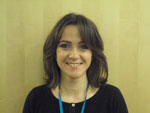
Myelodysplastic Syndromes (MDS) are a group of diverse and incurable pre-leukemic disorders. Even though a deregulated bone marrow microenvironment is thought to participate in the disease, its role remains elusive. Since the microenvironment is better understood in mice, we have identified a subset of cells in the human bone marrow that is equivalent to those cells in mice which, when perturbed, lead to MDS. We propose to characterize these cells in patient samples at the cellular and molecular level. This will provide a better understanding of the abnormal signaling pathways involved in MDS, and eventually identify therapeutic approaches.
Accomplished milestones
1- Confirm preliminary data on the human mesenchymal cell population corresponding to the mouse Osterix+ cells in the bone marrow that when perturbed induces myelodysplastic syndrome.
The fact that a genetic perturbation in a specific subset of osteoprogenitors is enough to induce an MDS-like disease in mice (Raaijmakers et al., 2010) highlights the importance of analyzing well defined and highly enriched subsets of cells when investigating abnormalities in the hematopoietic microenvironment. For this reason we sought to confirm our preliminary data on the identity of the human mesenchymal cell population corresponding to the mouse Osterix+ cells in the BM. We adopted a similar strategy of comparative gene expression analysis where we replaced microarrays with RNA sequencing which has an unlimited dynamic range allowing us to identify RNA activity at a much higher resolution which is important for detecting subtle differences in populations that are highly overlapping. For the isolation of mouse specific mesenchymal subsets, we used fluorescent genetic labeling where non hematopoietic and non endothelial GFP expressing cells under the control of either the Osterix (OSX) or the Osteocalcin (OCN) promoters were isolated by fluorescent activated cell sorting (FACS) and subjected to RNA sequencing in triplicates. In parallel, non hematopoietic, non endothelial human normal BM mesenchymal subsets were isolated by FACS using antibodies against CD-146, CD-271 and CD-106, that prospectively enrich for mesenchymal cell populations in human bone marrow (Mabuchi et al., 2013; Sacchetti et al., 2007) from three independent healthy donors. This strategy resolved four distinct populations: CD-271+CD-146+CD-106+ (DP), CD-271+CD-146+CD-106- (DN), CD-271+CD-106+ (SP) and CD-271+CD-106- (SN) (Figure1A) which were subjected to RNA sequencing. Functional analysis of differentially expressed gene sets using DAVID was performed to compare the DP, DN, SP and SN populations in humans and the OCN to the OSX population in mice. This has generated a list of significantly enriched functional terms as assessed by Benjamin-Hochberg false discovery rate (FDR). To correct for species differences that might account for low correlation values, we also performed functional analysis comparing CD- 45 and CD-51 populations in humans and mice since we know that these markers label similar hematopoietic and mesenchymal populations in both species. As expected, correlating functionally enriched terms in this comparison yielded the highest correlation values. Based on this we correlated the functionally enriched terms in the murine and human mesenchymal populations and the highest correlation value was obtained when comparing the SNvsDP populations (Figure 1B). We therefore concluded that A) SN is more mature, corresponding to OCN. B) DP is less mature, corresponding to OSX. Based on this and taking into consideration the relative abundance of each of the human mesenchymal populations we decided to analyze the following: DP, DN, SN+SP combined (S) in addition to the CD271-, CD146- (N) cells as a control since it is the least enriched population in mesenchymal stromal cells as assessed by colony forming unit formation.
2- Banking of MDS BM samples in addition to age and gender matched controls
To obtain primary samples of MDS bone marrow, we established collaborations with clinicians in major health care centers in the United States (Drs. Eyal Attar, David Sykes and Karen Ballen from Massachusetts General Hospital (MGH), Dr. David Steensma from Dana Farber Cancer Institute (DFCI), Dr. Omar Abdel Wahab from Memorial Sloan Kettering Cancer Center and Dr. Guillermo Garcia-Manero from MD Anderson Cancer Center In addition to a major international clinical center in China (Dr. Tao Cheng, Institute of Hematology in Tianjin). This center cares for large numbers of patients and has extensive experience obtaining clinical material for research. We visited the center in Tianjin and established an agreed upon standardized protocol for
banking, analyzing and sorting BM from MDS patients. As a result of these collaborations, we have been able to bank 56 MDS patient samples (Figure 2). Moreover, we have
been able to secure age and gender matched controls from patients undergoing hip replacement surgery.
3- Optimization of low input RNA sequencing protocol
Given the small volume of BM aspirate that we get from patients (1-5mls) and the low frequency of our characterized human OLC populations we anticipate a small yield of cells for analysis. For this reason, we have optimized and validated a protocol for library preparation and RNA sequencing of small numbers of cells ranging between 100-1000 per population. Quality control of the sequencing results demonstrated low intergenic and ribosomal bases in addition to a low duplication rate in all samples. On the other hand, the yield of mRNA and usable bases is as high as 73-75% indicating that we are able to capture a good amount of the transcriptome.
4- Phenotypic and gene expression analysis of MDS BM samples
We have currently completed the phenotypic analysis of 23 patient samples and 7 normal BM samples which did not demonstrate a significant difference in the frequency of the DP, DN or S population (Figure 3). All three populations in addition to the N population were sorted for RNA and DNA extraction. We have completed
RNA sequencing of 9 patient and 6 normal samples. For construction of the libraries, we used the Clontech SMARTer Ultra low input RNA system which were then sequenced on Illumina Hiseq2000 instrument, resulting in approximately 40 million paired-end 50 nucleotide reads per sample. The samples were aligned using STAR aligner and the QC metrics was generated using Picard tools. The libraries have low (Percentage of bases that pass filter/ Total number of bases) PCT intergenic bases averaging to ~ 0.15. The PCT ribosomal bases are also low (average ~ 0.013). The duplication rate averages about 36 % for all libraries. Libraries have good PCT mRNA bases and PCT usable bases, which are 0.656 and 0.652 respectively. The overall statistics indicate that we are able to capture good amount of transcriptome for all the samples analyzed.
Gene expression analysis identified sets of significantly differentially expressed genes within each population when comparing patient to normal: DP (48), DN (1019), S (1518), N (44) (Figure 4A). Interestingly, functional analysis of differentially expressed gene sets using DAVID resulted in significantly enriched terms that are unique to each population emphasizing the importance of analyzing specific cellular subsets as opposed to bulk populations (Figure 4B). Moreover, 8 out of the top ten functionally enriched terms within the S population are
genes involved in riobosome biogenesis (Figure 5). Genetic abnormalities that cause impaired ribosome biogenesis and function have been identified in a group of disorders known as riobosomopathies such as Diamond Blackfan Anemia and Schwachman-Diamond Syndrome (Narla and Ebert, 2010), however most of what has been described are abnormalities intrinsic to the hematopoietic cells. The only report indicating their implication in a cell extrinsic manner is by Raaijmakers et al. where the targeted deletion of Sbds, a gene encoding a protein that plays an important role in ribosome function and assembly, in OSX labeled osteoprogenitors resulted in an MDS like phenotype in mice (Raaijmakers et al., 2010). This emphasizes the validity of the approach we have adopted to identify deregulated pathway in the BM microenvironment of MDS patients.
Conclusion and future milestones
During the first year of my AAMDS grant, I was able to successfully bank 56 MDS samples through collaborations with major national and international clinical centers. Moreover, I was able to demonstrate the feasibility of analyzing specific BM stromal subsets that are rare for gene expression analysis by RNA sequencing. Data analysis of the first cohort of samples emphasized the validity of our approach. We are currently expanding the cohort of analyzed samples to be able to confirm our data. Meanwhile, we are running a more thorough analysis of the first cohort by comparing the differentially expressed genes to those that distinguish Dicer knock out OSX cells from wild type (Raaijmakers et al., 2010). Overlapping genes will be further validated using a co-culture platform of mesenchymal stromal layers and hematopoietic stem and progenitor cells (HSPCs) using a CRISPR-Cas9 based strategy to knock out candidate genes that are down regulated. We will be using a Cre recombinase dependent CRISPR-Cas9 knock in mouse (Platt et al., 2014) where CRISPR will be expressed in specific mesenchymal populations depending on the Cre line we use to generate the stromal layer for the co-culture. In the case of up regulated genes we will complement our CRISPR based knock out with an over expression strategy. Moreover, in addition to mesenchymal cells, we have been banking CD34+ HSPCs and BM plasma when possible from the same patients. We will use plasma to run cytokine arrays to validate any differentially expressed cytokines that emerge from our analysis. On the other hand, CD34+ cells will be used for gene expression analysis to investigate any changes that could be attributed to differentially expressed genes in the mesenchymal cells.
Bibliography
Mabuchi, Y., Morikawa, S., Harada, S., Niibe, K., Suzuki, S., Renault-Mihara, F., Houlihan, D.D., Akazawa, C., Okano, H., and Matsuzaki, Y. (2013). LNGFR(+)THY-1(+)VCAM-1(hi+) cells reveal functionally distinct subpopulations in mesenchymal stem cells. Stem Cell Reports 1, 152-165.
Narla, A., and Ebert, B.L. (2010). Ribosomopathies: human disorders of ribosome dysfunction. Blood 115, 3196-3205.
Platt, R.J., Chen, S., Zhou, Y., Yim, M.J., Swiech, L., Kempton, H.R., Dahlman, J.E., Parnas, O., Eisenhaure, T.M., Jovanovic, M., et al. (2014). CRISPR-Cas9 knockin mice for genome editing and cancer modeling. Cell 159, 440-455.
Raaijmakers, M.H., Mukherjee, S., Guo, S., Zhang, S., Kobayashi, T., Schoonmaker, J.A., Ebert, B.L., Al- Shahrour, F., Hasserjian, R.P., Scadden, E.O., et al. (2010). Bone progenitor dysfunction induces myelodysplasia and secondary leukaemia. Nature 464, 852-857.
Sacchetti, B., Funari, A., Michienzi, S., Di Cesare, S., Piersanti, S., Saggio, I., Tagliafico, E., Ferrari, S., Robey, P.G., Riminucci, M., et al. (2007). Self-renewing osteoprogenitors in bone marrow sinusoids can organize a hematopoietic microenvironment. Cell 131, 324-336.
Phenotypic and molecular characterization of osteolineage cells in patients with Myelodysplastic Syndrome.
Lay Summary
Blood has one of the highest tissue turnover rates, producing hundreds of billions of cells daily. This amazing productivity is maintained by a pool of hematopoietic stem cells (HSC), which is the most characterized adult stem cell. The ability of the HSC to both self renew and to contribute to all mature blood lineages is conserved by intrinsic as well as extrinsic cues. These extrinsic cues are provided by the bone marrow (BM) microenvironment, also known as the niche, which is a collection of cellular, physical and chemical factors. In the past five years, evidence has unfolded implicating the niche in the pathogenesis of several hematological disorders including myelodysplastic syndromes (MDS).
Myelodysplastic syndromes are a diverse group of hematological disorders affecting primitive hematopoietic cells resulting in inefficient hematopoiesis and the risk of transformation to leukemia. Despite the introduction of several drugs for the treatment of MDS, the only curative therapy remains that of allogeneic stem cell transplant (allo-SCT). The allo-SCT is not the treatment of choice for many MDS patients given the very high risks of treatment-related morbidity and mortality. That being said, there is an urgent need for the development of new innovative therapies based on a thorough understanding of the molecular mechanisms underlying MDS.
Several genetic abnormalities have been identified in malignant hematopoietic MDS cells and the mechanisms of their contribution to MDS pathogenesis is an area of ongoing research. However, even though a role of a deregulated microenvironment has long been postulated to contribute to the complex pathology underlying MDS, these mechanisms remain elusive. Recent scientific evidence in animal models supports the role of the microenvironment in the maintenance and even in the initiation of the disease, however, strong evidence from patients is still lacking. Earlier attempts to tackle this issue were hampered by our poor understanding of the hematopoietic microenvironment in humans and by the lack of tools to characterize it. For this reason, studies have been performed under suboptimal conditions, relying on characterizing artificial cell populations expanded outside of the body and likely not a good representation of what is happening in patients.
Through a generous grant from the Aplastic Anemia and MDS foundation we were able to better understand what cell populations constitute the human BM hematopoietic niche and to develop methods that allowed us to characterize them in the context of patients with MDS. As a result we have now generated a database of molecular abnormalities that we are using as a platform for the design of therapeutic strategies for MDS.
Accomplished milestones 2015
1- Define the human mesenchymal cell population corresponding to the mouse Osterix+ cells in the bone marrow that when perturbed induces myelodysplastic syndrome.
We have used fluorescent genetic labeling in mice to distinguish OSX labeled osteoprogenitors from OCN labeled osteoblasts. Cells were isolated by fluoresence activated cell sorting (FACS) and subjected to RNA sequencing. In parallel, in order to identify the human mesenchymal population that is equivalent to the OSX labeled one in mice, we have used a combination of three cell surface markers (CD-271, CD-146 and CD-
106) that have been previously shown to prospectively enrich for self-renewing mesenchymal cells within the human bone marrow [2-4]. Analysis of samples obtained from bone marrow filter bags from eligible bone marrow donors using antibodies against the previously mentioned cell surface markers within the hematopoietic and endothelial negative compartment as labeled by (CD-45, CD-235, CD-34 and CD-31) resolved four distinct populations CD-271+CD-146+CD-106+ (DP), CD-271+CD-146+CD-106- (DN), CD-271+CD-106+ (SP) and CD-271+CD-106- (SN) (Figure 1 A) which all had a frequency ranging from 0.01-0.02% (Figure 1 B) . Compared to CD-271-CD146- (N) cells, the DP population had the highest number of colony forming units (CFU-F) as reflected by the fibroblastic colony forming unit assay indicating an ability to self-renew (Figure 1C). Moreover, the DP population demonstrated a robust ability to differentiate in vitro into osteogenic, adipogenic and chondrognic lineages (Data not shown) in addition to supporting hematopoietic stem and progenitor cells in vitro (Figure 1D) and forming ossicles under the skin of immuno-compromised mice.
We then subjected the murine and human mesenchymal populations described above to RNA sequencing. Functional analysis of differentially expressed gene sets using DAVID was performed to compare the DP, DN, SP and SN populations in humans and the OCN to the OSX population in mice. This has generated a list of significantly enriched functional terms as assessed by Benjamin-Hochberg false discovery rate (FDR).
To correct for species differences that might account for low correlation values, we also performed functional analysis comparing CD-45 and CD-51 populations in humans and mice since we know that these markers label similar hematopoietic and mesenchymal populations respectively in both species (Figure 2A). As expected, correlating the expression of genes contributing to the functionally enriched terms in this comparison yielded the highest correlation values (Figure 2B). Based on this we correlated gene expression within the functionally enriched terms in the murine and human mesenchymal populations and the highest correlation value was obtained when comparing the SNvsDP populations (Figure 2B). We therefore concluded that A) SN is more mature, corresponding to OCN. B) DP is less mature, corresponding to OSX. Based on this and taking into consideration the relative abundance of
each of the human mesenchymal populations we decided to analyze the following: DP, DN, SN+SP combined (S) in addition to the CD271-, CD146- (N) cells as a control since it is the least enriched population in mesenchymal stromal cells as assessed by colony forming unit formation.
2- Optimization of a low input RNA sequencing protocol
Given the small volume of BM aspirate that we get from patients (1-5mls) and the low frequency of our characterized human mesenchymal populations we anticipated a small yield of cells for analysis. For this reason, we have optimized and validated a protocol for library preparation and RNA sequencing of small numbers of cells ranging between 100-1000 per population. Quality control of the sequencing results demonstrated low intergenic and ribosomal bases in addition to a low duplication rate in all samples. On the other hand, the yield of mRNA and usable bases is as high as 73-75% indicating that we are able to capture a good amount of the transcriptome.
3- Phenotypic analysis of MDS BM mesenchymal populations
We completed the phenotypic analysis of 23 patient samples and 7 normal BM samples which did not demonstrate a significant difference in the frequency of the DP, DN or S population (Figure 3).
Figure 3: Frequency of the different stromal populations within the non-hematopoietic, non-endothelial BM cells
Accomplished milestones 2016
1- Molecular analysis of MDS BM mesenchymal populations
The DP, S, DN and N populations were sorted and subjected for RNA sequencing from a total of 11 normal donors and 14 MDS patients from which we were able to get enough cells for the analysis (Figure 4). The overall statistics indicate that we are able to suffiiently capture a good amount of the transcriptome for all samples analyzed. Principal component analysis revealed that the transcriptional profile of normal samples clearly separated from MDS patients within all analyzed populations with the S population demonstrating the most distinct clustering (Figure 5A). Gene expression analysis identified sets of significantly differentially expressed genes within each population when comparing patient to normal samples including genes encoding for secreted molecules, cell surface markers, transcription factors, and kinases (Table 1). Of note, functional analysis using DAVID identified terms involved in ribosome biogenesis, protein translation, and extracellular matrix (Figure 5B). Moreover, overlap between functionally enriched terms among the four populations was minimal indicating a unique signature of each population (Figure 5C).
2- Molecular analysis of CD34+ hematopoietic cells from paired normal and patient bone marrow
Complimentary to the analysis of the mesenchymal populations, we have analyzed paired BM CD34+ cells from 6 normal donors and 11 patients by RNA sequencing. Through pathway and receptor ligand analysis we are investigating how the transcriptional abnormalities in the mesenchymal cells are affecting the hematopoietic cells and hence the pathogenesis of the disease. Preliminary analysis of this data shows a clear separation between patient and normal samples. Moreover, CD34+ cells show a distinct expression profile when compared to the mesenchymal cells from the same donor ruling out contamination with hematopoietic cells.
Conclusion and future perspectives
Our data demonstrate the transcriptionally distinct subsets of BM mesenchymal cells and identify signatures of transcriptional deregulation which differentiate these cell subsets in MDS patients from normal individuals. These data represent a valuable resource for identifying molecules that could be therapeutically targeted. Moreover, the results emphasize the importance of analyzing specific cellular subsets as opposed to bulk populations and the validity of the approach we have adopted to identify deregulated pathways in the HMEV of MDS patients.
Based on the generated data, we are currently investigating potential therapeutic targets among the perturbed genes mainly the over expressed ones through co-culture assays in addition to the humanized niche ossicle model in immunocompromised mice
Bibliography
1. Raaijmakers, M.H., et al., Bone progenitor dysfunction induces myelodysplasia and secondary leukaemia. Nature, 2010. 464(7290): p. 852-7.
2. Sacchetti, B., et al., Self-renewing osteoprogenitors in bone marrow sinusoids can organize a hematopoietic microenvironment. Cell, 2007. 131(2): p. 324-36.
3. Tormin, A., et al., CD146 expression on primary nonhematopoietic bone marrow stem cells is correlated with in situ localization. Blood, 2011. 117(19): p. 5067-77.
4. Mabuchi, Y., et al., LNGFR(+)THY-1(+)VCAM-1(hi+) cells reveal functionally distinct subpopulations in mesenchymal stem cells. Stem Cell Reports, 2013. 1(2): p. 152-65.
5. Narla, A. and B.L. Ebert, Ribosomopathies: human disorders of ribosome dysfunction. Blood, 2010.
115(16): p. 3196-205.